Samples are currently making news for NASA’s planetary exploration program. Last August, the rover
Curiosity, equipped with a package of laboratory instruments, landed on Mars. On February 9th the rover’s robotic arm
drilled its first hole in a rock selected by scientists. In their attempt to gain more information about Mars, scientists will use the rover’s science package to remotely analyze these samples on the martian surface. The results will give them some fairly detailed knowledge on the chemical and mineral make up of these rocks. But what else can we possibly learn from samples?
Geologists in general and planetary scientists in particular often emphasize that “such and such” cannot be known for certain “until we obtain samples” of some planetary surface or outcrop. What is this obsession with samples? Why do (some) scientists value them so highly and exactly what do they tell us? Answers to this question (for there is not a single, simple one) are more involved than you might think.
With today’s technology providing us with only the most rudimentary information, sample analyses made remotely on a distant planetary surface is limited. Some of the things we want to know, such as the formation age of rocks, can only be discovered with high precision, careful laboratory work. That’s a tall order for remote systems. For example, one of the most common
techniques used to “date” a rock’s age requires the separation of individual minerals that make up the rock. Next, the ratio of minute trace elements and their isotopes in each grain must be determined. Assuming that the rock has not been disturbed by heating or a crater shock event, this information can be used to infer an age of formation. If we can convince ourselves that the rock being studied is representative of some larger unit of regional significance, we can use this information to reconstruct the geological history of the region and eventually, the entire alien world. So sample analysis is an important aspect of geological exploration.
As I have
written previously, we used images to geologically map the entire Moon, noting its crater, basin and mare deposits, and their relative sequence of formation. When the first landing missions were sent to the Moon, great emphasis was placed on obtaining representative samples of each landing site. It was thought that such samples could be studied in detail in Earth laboratories and then extrapolated to the larger regional units shown on the geologic maps. With few exceptions, this approach worked pretty well. As we moved from the landing sites on the maria (ancient lava flows) into the complex highlands, the “context” of the samples – their relation to observed regional landforms or events – became more obscure. A lunar highland rock is typically a complex mixture of earlier rocks, sometimes showing evidence for several generations of mixture, re-fragmentation, and re-assembly. Loose samples lying on the surface were collected from the highlands, none of them were sampled “in place” (i.e., from bedrock). Although this is also true of the rocks from the maria, we observed bedrock “in place” at most of the mare sites and may have actually collected at least one sample from lava bedrock at the edge of
Hadley Rille near the Apollo 15 site.
None of the highland samples possess the same degree of contextual certainty as the mare samples. This fact, coupled with their individual complexity, sometimes leads to consternation over exactly what the samples are telling us. It doesn’t help that the Moon’s early history was itself very complex, with magmas solidifying, lavas erupting, volcanic ash hurled into space and laid down in bedded deposits. On top of all those processes were cratering events that mixed and reassembled everything into a complex geologic puzzle, a virtual stew of processes and compositions that hold clues to billions of years of the Moon’s (and Earth’s) history. Nonetheless, we can still perceive most of the story of the Moon’s history, enough at this point to tell us that without those lunar samples in hand, we would be well and truly ignorant of even its most important events and basic processes. The fixation with sample return stems from the science community’s belief that with just a few more carefully selected samples from some key units, all that is now dark will be made light.
There may be severe consequences to the science community’s insistence on the primacy of sample return. The most recent “decadal survey,” the ten-year community study that gives NASA our wish lists for missions and exploration,
made a sample return from Mars the centerpiece and
sine qua non of future robotic missions. The
NRC report was so emphatic in its insistence that it might be paraphrased as saying, in effect, “Give us a Mars sample or give us death!” (with apologies to Patrick Henry). Alas, that formulation may be more apt than anyone desired, as proposed out year budgets for the next five years of NASA funding cuts planetary exploration by almost 30% – a landscape of shifting priorities that raises questions and uncertainty for the future.
Robotic sample return missions to large bodies like the Moon or Mars are expensive because they consist of multiple spacecraft – a lander, which softly places the spacecraft on the surface, a device (such as a rover) to collect and store the samples and an ascent vehicle to bring the sample back to Earth. While none of these functions individually are exceedingly difficult to achieve, all of them (done correctly and in proper sequence) add up to a substantially difficult, complex mission profile.
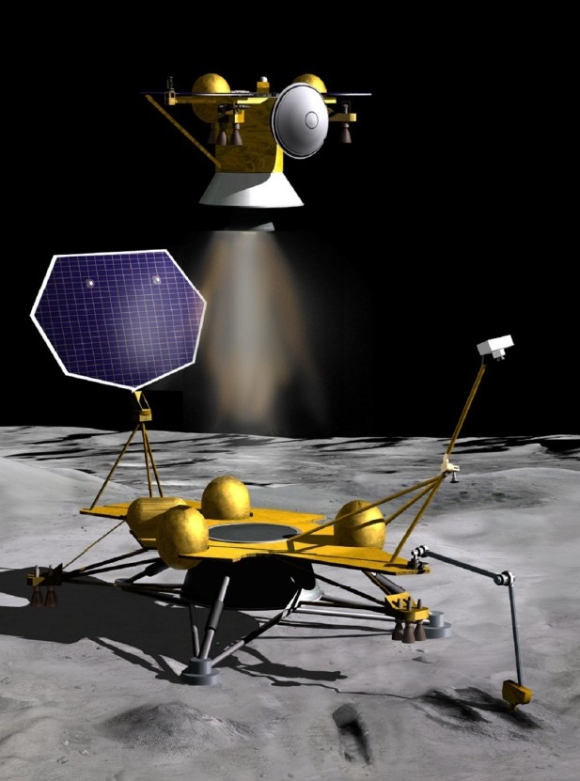 |
Among the more recent artist's representation of the high-priority MoonRise mission, ascending from South-Pole Aitken basin [NASA/JPL]. |
In the space business (as with most endeavors), more difficult and complex means that more money is required.
Moonrise, a proposed robotic mission to return about a kilogram of sample from the far side of the Moon, was projected to cost around one billion dollars. A Mars sample return mission consisted of three separate missions: one to land, collect and store the samples, another one to retrieve those samples and place them into orbit around Mars, and a final mission to return the samples to Earth. With each step costing up to several billion dollars, such a technically challenging Mars sample return mission would be unaffordable.
Although samples have many advantages over remote measurements, those benefits must be weighed against the cost and difficulty of obtaining them. Perhaps the complete extent of what can be accomplished remotely has yet to be fully explored. As mentioned above, absolute ages are key information that we get from samples. Several dating techniques could be adapted to a remote instrument; these methods may not be the most precise imaginable, but they might be of adequate precision to answer the most critical questions. On the Moon, we do not know the absolute age of the youngest lava flows in the maria; age estimates range from as old as ~ 3 billion years to as young as less than 1 billion years. In such a case, a measurement with 10-20% precision is adequate to resolve the first-order question: When did lunar volcanism cease? In addition, such a result would enable us to calibrate the cratering curve for this part of lunar history, a function that is widely used to infer absolute ages throughout the Solar System. A solid result obtained from a robotic lander – even such a relatively imprecise one – would have important implications for lunar volcanic processes, thermal history, impact flux, and bulk composition.
Complex robotic operations in space are always dicey, especially when attempting something for the first time. Samples are a key part of a planetary scientist’s toolbox but their acquisition is difficult, time-consuming and expensive. Samples from robotic missions are more likely to have ambiguous context, thus rendering less scientific value. Scientifically useful sample collection may remain problematic until people can physically go to exotic places in space and fully use their complex cognitive skills. This trade-off between cost and capability must be carefully considered when weighing future exploration alternatives and desired outcomes.
Previous Relevant Posts:
Humans and field work
Lunar robotic sample return
Mars sample return
The Last Sampler: Failure, then Success
India hopes to join JPL Moonrise mission to SPA
Moonrise SPA sample-return mission and Washington University