Monday, January 31, 2011
IAU names craters to honor Columbia crew
Saturday, January 29, 2011
New Robotic Lander Prototype skates tests
Kim Newton
Marshall Space Flight Center
The lander prototype was placed on modified skateboards and a customized track system as a low-cost solution to control movement during final testing of the prototype’s sensors, onboard computer, and thrusters. The functional test focused on ensuring that all system components work seamlessly to sense, communicate, and command the lander's movements.
The prototype will be transported to the United States Army Redstone Arsenal Test Center in Huntsville this week to begin strap-down testing, which will lead to free-flying tests later this year.
The lander prototype will aid NASA’s development of a new generation of small, smart, versatile landers for airless bodies such as the moon and asteroids. The lander's design is based on cutting-edge technology, which allows precision landing in high-risk, but high-priority areas, enabling NASA to achieve scientific and exploration goals in previously unexplored locations.
Development of the lander prototype is a cooperative endeavor led by the Robotic Lunar Lander Development Project at the Marshall Center, Johns Hopkins Applied Physics Laboratory of Laurel, Maryland and the Von Braun Center for Science and Innovation, which includes the Science Applications International Corporation, Dynetics Corporation, Teledyne Brown Engineering, Inc. and Millennium Engineering and Integration Company, all of Huntsville.
For more information on the Robotic Lunar Lander Development Project, please visit http://www.nasa.gov/roboticlander.
Friday, January 28, 2011
The Challenger Crater Group of Apollo Basin
Mark Robinson
Principal Investigator
Lunar Reconnaissance Orbiter Camera
Arizona State University
After the loss of the Space Shuttle Challenger these seven craters on the eastern rim of Apollo were named after Greg Jarvis, Christa McAuliffe, Ron McNair, Ellison Onizuka, Judy Resnik, Dick Scobee and Mike Smith.
View the WAC mosaic of the entire Apollo basin and surroundings.
Visit NASA's Day of Remembrance webpage, HERE.
NASA Lunar Science Forum IV
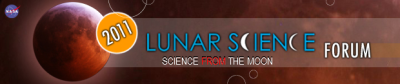
FIRST ANNOUNCEMENT:
Clive R. Neal
University of Notre Dame
This year's forum will feature sessions on recent scientific results from the Lunar Reconnaissance Orbiter (LRO) and Lunar Crater Observation and Sensing Satellite (LCROSS), dedicated side-conferences for graduate students and young lunar professionals, as well as the annual recognition of scientific accomplishments and associated keynote lecture.
As in past years, science sessions are structured to report on both recent results and future opportunities for lunar science, exploration, education and outreach.
We also look forward to news on the upcoming lunar missions GRAIL and LADEE and welcome abstracts across the many fields of lunar science.
Abstracts will be accepted starting February 21 through May 2, 2011 at http://lunarscience.nasa.gov/lsf2011
February's announcement will discuss the Lunar Science Forum logistics, but please save the date now as you make your summer meeting plans.
We look forward to another exciting meeting focusing on science Of, On and From the Moon!
Note: The 4th Annual NLSI Conference will be held once again this year at the NLSI's host facility, NASA's Ames Research Center at Moffett Field, California.
Rille in Aitken Crater
Drew Enns
LROC News System
Search for more interesting features in the NAC frame.
Related Posts
Terraced Craters in Aitken Crater
Exposed Boulders in the Aitken Mare
Exposed Boulders in the Aitken Mare
Drew Enns
LROC News System
Can you find more boulders in the NAC image?
Related Posts:
Wrinkle Ridges in Aitken Crater
Terraced Craters in Aitken Crater
Gassendi's Fractures
Bouncing, Bounding Boulders
Tuesday, January 25, 2011
Terraced Craters in Aitken Crater
Drew Enns
LROC News System
Find more craters in the full NAC frame!
Related Posts:
Wrinkle Ridges in Aitken Crater
Aitken Central Peak, Seen Obliquely
Approaching Aitken Crater - Vertregt J
Saturday, January 22, 2011
Wrinkle Ridges in Aitken Crater
Drew Enns
LROC News System
Search for more wrinkle ridges in the mare and highlands in the whole NAC mosaic.
Related Posts:
Aitken's Central Peak, Seen Obliquely.
Thursday, January 20, 2011
Vertregt J: Approaching Aitken
Mark Robinson
Principal Investigator
Lunar Reconnaissance Orbiter Camera
Arizona State University
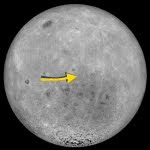
As it turns out, results from the Clementine and Lunar Prospector missions showed lunar scientists that the chemistry of Apollo 16 rocks differs significantly from most the highlands. Murphy's law at work!
Lunar scientists need samples from other highland targets, especially inside the South Pole Aitken Basin (SPA) basin, to get a better handle on the origin of the lunar crust and the history of asteroid bombardment early in our solar system's history.
Explore at full resolution the Vertregt J oblique view.
Explanation of "lettered craters" on the Moon.
Tuesday, January 18, 2011
Oblique view of Aitken's central peak
Mark Robinson
Principal Investigator
Lunar Reconaissance Orbiter Camera
Arizona State University
This latest LROC oblique view gives you a sense of what astronauts will see on their terminal descent into Aitken. Check out the stunning full-resolution image and think about where you would go inside this spectacular geologic feature!
Read some of our previous postings about Aitken crater here, here, and here! And visit the central peak of Bhabha crater.
Friday, January 14, 2011
HEFT, Lies and Videotape
Paul D. Spudis
The Once & Future Moon
Smithsonian Air & Space
A real comedy of errors and misunderstandings collided this week between the new NASA Authorization Act of 2010 and the agency’s Human Exploration Framework Team (HEFT) Congressionally mandated 90-day report (their initial findings on how to implement agency direction). Thought flush with the usual beautiful graphics and platitudes, the report’s bottom line is that under the existing budget and schedule, the agency cannot make the new heavy lift launch vehicle specified in the new authorization bill. In other words, you can’t get there from here. Can’t be done. Period.
Reading through the new report is an exercise in déjà vu for space policy geeks. It reads very much like the Exploration Systems Architecture Study (ESAS) of 2005. No big surprise, when one realizes that many of the people who wrote that report are involved in the new one. But more than that, the sense of a previous life stems from the rocket design that has resulted from this effort. It looks remarkably similar to the rocket that resulted from the previous effort, the late and not-so-lamented Ares family of heavy lifters. As you may recall, a key conclusion of the oft-cited Augustine Committee report was that the existing program of record (Project Constellation, a.k.a. Ares rocket) was unaffordable without infusions of significant quantities of new money.
Did NASA get a big infusion of cash? No. So no one should be surprised that the same people, working under the same assumptions within the same agency and technology base as the Project Constellation people would reach the same conclusions. In fact, most were not surprised. But apparently, many in the United States Senate did expect a different answer. Or did they?
We now enter the political Hall of Mirrors in which what people say they want isn’t necessarily related to what they really want or don’t want. Let us see if we can chart a path through the maze of motives, desires and statements to fully understand exactly what’s going on. Please stay with me on this until the end; I will try to make things clear.
Seven years ago, we had the Vision for Space Exploration (VSE), a statement of strategic direction in space. The VSE called for returning Shuttle to flight after the Columbia accident, completing construction of the ISS, the building of a new space transportation system, a return to the Moon (“with the goal of living and working there for increasing periods of time”) and finally, human missions to Mars and “other destinations.” After the VSE was announced, NASA “implemented” it by completing steps 1 and 2. Step 3 was started, outlining an architecture and design for a new human spacecraft and new launch vehicles (the ESAS). We never progressed beyond that, although many departments, universities and international partners dug in and began conducting studies of work and instruments needed to live on the Moon.
It is a fool’s errand to design architectures and new space vehicles if you do not know what your mission is. You can design and build a space system without an objective but as it must satisfy many different purposes, it tends to not satisfy any of them particularly well. From the beginning, NASA leadership didn’t acquaint itself with why they were tasked with lunar return, even though the VSE founding documents are quite clear on the purpose and activities associated with lunar return. Because of this strategic confusion, it was largely assumed by many that we would do on the Moon what we did 40 years ago – explore, collect samples, and leave as soon as possible (that last activity being particularly favored within the agency). To accommodate this activity, the Ares launch vehicles were designed to conduct a lunar mission with two launches – the Ares I, which would put the crew vehicle in low Earth orbit and the Ares V, which carried all the other pieces. Additionally, NASA never lost sight of its desire for Mars, so Ares V was sized at a payload capacity of 160 tons, overkill for a lunar mission but thought to be the right number for a human Mars mission, staged completely from the surface of the Earth (whether that’s true is another story).
As Ares rocket development costs rose, other pieces of the lunar return architecture were discarded. Eventually, we had a large rocket-building program but its purpose had become diffuse and nebulous (in 2009, the acting Administrator of NASA told Congress in testimony that he did not know what going to the Moon meant).
Curiously, the new NASA Authorization Act of 2010 was remarkably specific about the requirements of a new heavy lift vehicle the agency had been directed to build. It was to use Shuttle hardware to “the extent practicable” and initially carry 70-100 tons but designed such that it could be stretched to a lift capacity of 130 tons. Where did these numbers come from? It’s not clear, but here’s an interesting coincidence: 130 tons was the lift the capacity of the old Saturn V (118,000 kg = 260,000 pounds = 130 tons). NASA has interpreted the new Congressional language as meaning metric tons (2200 lbs) but the simple language of the law says “tons” (1 ton = 2000 lbs). One might suspect that the calculus was that heavy lift in days of old (Saturn V) meant 130 tons, so that’s what “heavy lift” should be.
In the absence of any specific mission, the payload capacity of your launch vehicle is entirely academic. But this “requirement” has had some serious ramifications. Last summer, a study group at Johnson Space Center released a report (Preliminary Report Regarding NASA’s Space Launch System and Multi-Purpose Crew Vehicle, Pursuant to Section 309 of the NASA Authorization Act of 2010 (P.L. 111-267), SSP Study NSTS 60583, dated June 8, 2010) showing how a heavy lift vehicle could be built and flown under the then-current run out budget (any new budget for NASA is pure guesswork at this stage). It resurrects an old concept of replacing the Shuttle orbiter on the existing stack with a payload fairing and engine pod. This configuration, called Shuttle Side-Mount (updated from the old “Shuttle-C” concept) was not considered by the HEFT study team, but meets the specific language of the new authorization. The advantage of SSM is that, as it is a minimal modification of the existing stack, it uses all of NASA’s existing launch and processing infrastructure – launch pads, mobile crawlers, scaffolding in the VAB and fabrication facilities in Michoud and Utah. SSM initially carries about 80 metric tons (70 (63.3 metric) to 100 (90.7 metric) tons) and can be stretched to meet the 130 ton (118 metric tons) legal requirement with minimal modification (for example, adding 5-segment (instead of 4-segment) Solid Rocket Boosters, 4 Shuttle Main Engines, extended External Tank). So in fact, SSM meets all the technical, budgetary, safety and schedule requirements set out in the NASA Authorization Act of 2010.
So as Oliver Hardy would say, here’s another fine mess we’ve gotten ourselves into. NASA creates an unaffordable architecture (ESAS) to implement the VSE. The response by the new administration is to cancel the VSE and replace it with promises of more distant goals at some nebulous time in the far future. Congress directs the agency to build an HLV anyway, but the vehicle has no mission, so they pull out the specs of the last HLV America flew. NASA responds by saying they can’t do it on the money and schedule specified, even though they themselves have in hand a report that shows how it can be done. Moreover, the agency still claims it doesn’t know why anyone would want to go to the Moon, despite having been shown repeatedly that what we do there will create new space faring capability.
You just gotta love this business.
Tuesday, January 11, 2011
Moon Flower
Saturday, January 8, 2011
Rep. Giffords critically injured in shooting

Jared Loughner (b. 1988) has been arrested in connection with the shooting.
Irresponsible rumors, including deliberate disinformation, about possible political motives for the shooting are flying back and forth, but one friend and fellow "former band member" Caitie Parker of Ohio Valley in Arizona describes the alleged shooter as "reclusive" since alcohol poisoning in 2007.
She described his politics as "definitely left wing," though most sources demonstrate the assailant to be mentally ill.
Thursday, January 6, 2011
Regolith: The "Other" Lunar Resource
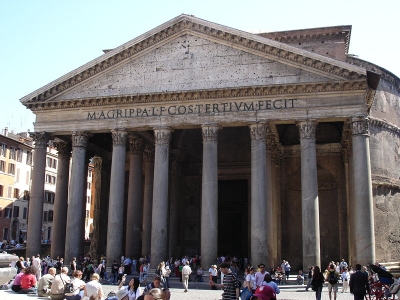
The Pantheon of Rome, a 2000-year old concrete structure.
Paul D. Spudis
The Once & Future Moon
Smithsonian Air & Space
In civil engineering, one of the most important material resources on Earth is “construction aggregate” – the sand, gravel and cement building materials that make up the infrastructure of modern industrial life. Aggregate is easily one of the biggest, most valuable economic resources of all mined terrestrial materials – more so than gold, diamonds, or platinum. We depend on aggregates for many different types of objects; they are the fundamental building block of roads and structures. The use of aggregates in building goes back to ancient civilizations; concrete was used in buildings of ancient Egypt. The Romans devised a recipe for a concrete so durable that the molded arches, walls and self-supporting dome of the Pantheon (made over 2000 years ago) stand today. Aggregates in terrestrial use typically depend on a lime-based cement that bonds the particulate material together. Both lime (CaO) and abundant water are needed to make concrete on Earth.
On this blog and elsewhere I have detailed the importance and significance of water at the poles of the Moon. Water is indeed the most important early product to produce from lunar materials but there are other resources on the Moon. A permanent presence on the Moon will require infrastructure that must by necessity use as much local material as possible. Aggregate materials probably will become the primary building blocks of industrial society off planet, just as it has on the Earth. The composition and conditions of local materials will require some adjustments as to how we use lunar aggregate. A little thought reveals some interesting parallels and differences with terrestrial use.
On Earth, gravel pits are carefully located to take advantage of the sorting and layering produced by natural fluvial (river water-eroded) activity. We harvest gravels from alluvial plains and old river beds, where running water has concentrated rocks, sand and silt into deposits that can be easily excavated, loaded, and transported to sites of construction. The highly variable currents, as well as the velocities of flow of our terrestrial streams and rivers, sort the aggregate by size, creating layers of gravel-sized up to cobble-sized stones for the fastest flowing waters. Finer grained material is likewise concentrated where water speeds are low and sand and silt settles out from the suspended sediment (the “bed load”).
No natural process on the Moon creates such deposits, but the lunar surface rock has already been disaggregated by impact into a chaotic upper surface layer called regolith. Regolith is basically ground-up bedrock; impacts of all sizes constantly pummel the surface, breaking, fracturing and grinding up the Moon’s bedrock. Impact both breaks up and creates rock. An impact will destroy a rock both by shock (catastrophic rupture) and through cratering (fragmentation and excavation). The effect of such destruction is to make “soil,” fine-grained rocky material made up of the mineral grains of the bedrock. But impact also creates heat and this heat can weld small fragments into glass-rich aggregate rocks (regolith breccias) as well as quickly cooled fragments of melt that contain mineral inclusions (agglutinates, or glass). In broad terms, impacts destroy and disaggregate more than they create and weld together. Thus, on a given surface, regolith thickness increases with time – older surfaces have thicker regoliths.
The ground up regolith is a readily available building material for construction on the lunar surface. It is an aggregate in the same sense as on Earth, but with some significant differences. We could make lime and water from the surface materials of the Moon but it is very time and energy intensive. Thus, we must adapt and modify terrestrial practice to take advantage of the unique nature of lunar materials. The fractal grain size in the regolith means that we can obtain any specific size fraction we want through mechanical sorting (raking and sieving). Instead of water-set lime-based cement, we can use glass to cement particulate material together. Regolith can be sintered into bricks and blocks, as well as roads and landing pads, using thermal energy (passive solar, concentrated by focusing mirrors) or microwaves that can melt grain edges into a hard, durable ceramic.
The use of aggregate materials on the Moon will likely be gradual and incremental. Our initial presence on the Moon will be supported almost entirely by materials and supplies brought from Earth. As we gain facility using lunar resources, we can incorporate more and more local materials into structures. Simple, unmodified bulk soil is an early useful product. It can be used to build berms to protect an outpost from the rocket blast of arriving or departing spacecraft and to cover surface assets for thermal and radiation protection. The next phase will be to pave roads and pads to keep down randomly thrown dust and provide good traction for the multitude of wheeled vehicles supporting the outpost. Fabrication of bricks from regolith will allow us to construct large buildings, initially consisting of open, unpressurized workspaces and garages but ultimately, habitats and laboratories. Making glass by melting regolith can produce building materials of extreme strength and durability; anhydrous glass made from lunar soil is stronger than alloy steel with a fraction of its mass.
Eventually, we may be able to export these lunar building materials into space. A major drawback is the gravity well of the Moon – its escape velocity is about 2.38 km/s, smaller than that of the Earth but substantial. To use large quantities of lunar materials for space construction, we need to develop an inexpensive means to get material off its surface. Fortunately, the small size and no atmosphere of the Moon make this possible by literally throwing stuff off the Moon into space. A “mass driver” can launch objects off the lunar surface by accelerating them along a rail track using electromagnetic coils that hurl capsulated material into space at specific velocities and directions. We can collect such thrown material at a convenient location, such as one of the libration points. From there, it is a relatively simple matter to send the material to wherever it is needed in cislunar space.
Water remains the most important first lunar product, but the “other” lunar material regolith is almost as important. Lunar rock and soil will be the paving stones of the Solar System. As once all roads led to Rome, all new roads in cislunar space lead to – and from – the Moon.
Monday, January 3, 2011
Quanrantid stream followed by partial eclipse
![]() |
From 41st Lunar & Planetary Science Conference (2010) |
In western Europe, as much as 86% of the solar disk will be covered by the Moon at dawn, producing a fantastic crescent sunrise on Jan. 4th. Follow the links for a live webcast, an animated map, and details from NASA