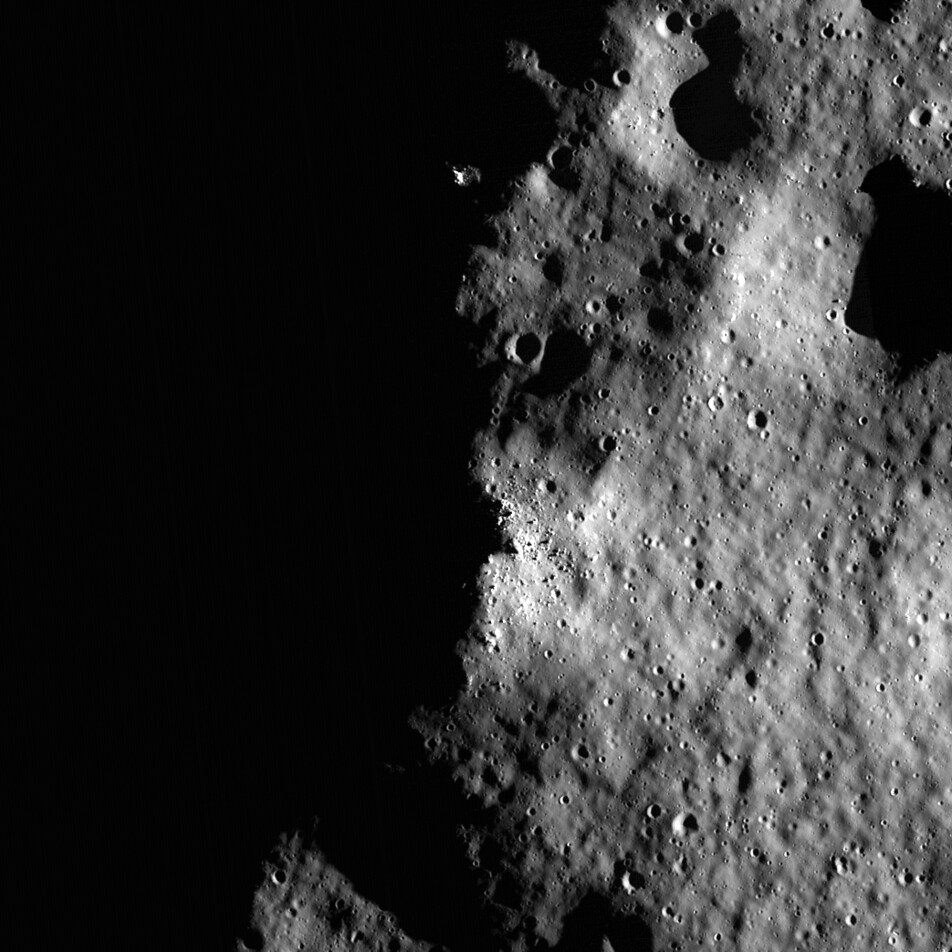 |
Close up on the rim of Shackleton crater, a portion of the first LROC image in lunar orbit, released as LROC Featured Image on the Fifth Anniversary of "First Light," July 3, 2014. This region of the rim of Shackleton, near the lunar South Pole, is illuminated over 70% of the time, while the floor of the crater is permanently shadowed. LROC NAC mosaic M101013931LR, LRO orbit 72, June 30, 2009; 90.01° incidence angle, resolution 1 meter from 42.96 km over 89.38°S, 53.48°E [NASA/GSFC/Arizona State University]. |
LROC News System
The
first image captured by LROC from lunar orbit was acquired on 30 June 2009.
When the LROC team first glimpsed that image, some twelve days after LRO’s launch from Cape Canaveral, there was a mix of pride that all of the hard work to build and calibrate LROC was coming to fruition, relief that the cameras were in perfect working order, and anticipation for the adventures to come.
Now, on the fifth anniversary of LROC’s first image, we examine it again, with the insights that come with five years of knowledge gained.
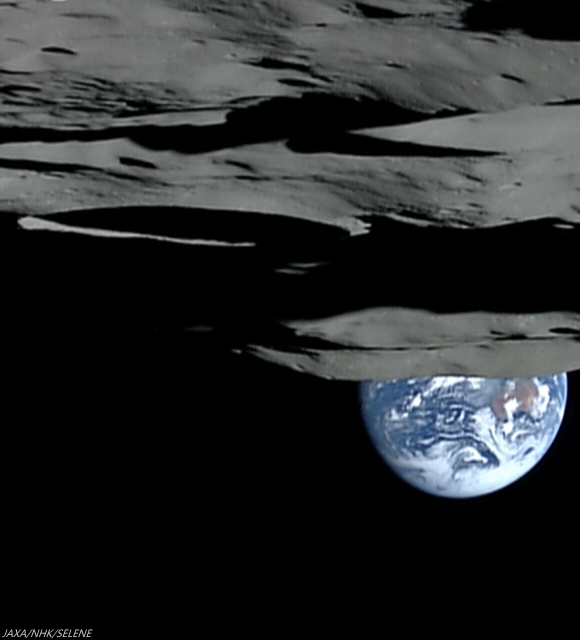 |
An Orbital Earthset, from Japan's lunar orbiter Kaguya, November 2007, behind Malapert massif, and 10 km-wide Shackleton crater, both features of the Moon's far south visible from Earth at favorable librations. Between them, in permanent shadow, like the interior of Shackleton, is invisible Shoemaker crater, where a signature of a kind of volatile frost, perhaps composed of water, has been detected [JAXA/NHK/SELENE]. |
As it happens, this first image shows off one of the most interesting lunar science and exploration sites, Shackleton crater. Shackleton is located at the Moon’s
South Pole, with a portion of its rim just grazing 90° south latitude. One of LROC’s
primary objectives, defined as the mission was being conceived, is to map regions of permanent shadow or permanent illumination.
Because the Moon is just barely tilted on its spin axis (1.5 degrees compared to the 28.5 degrees that give us our seasons on Earth), any low point near the pole has the potential to be forever shaded from the Sun and peaks could forever poke above the horizon to catch grazing rays from the Sun.
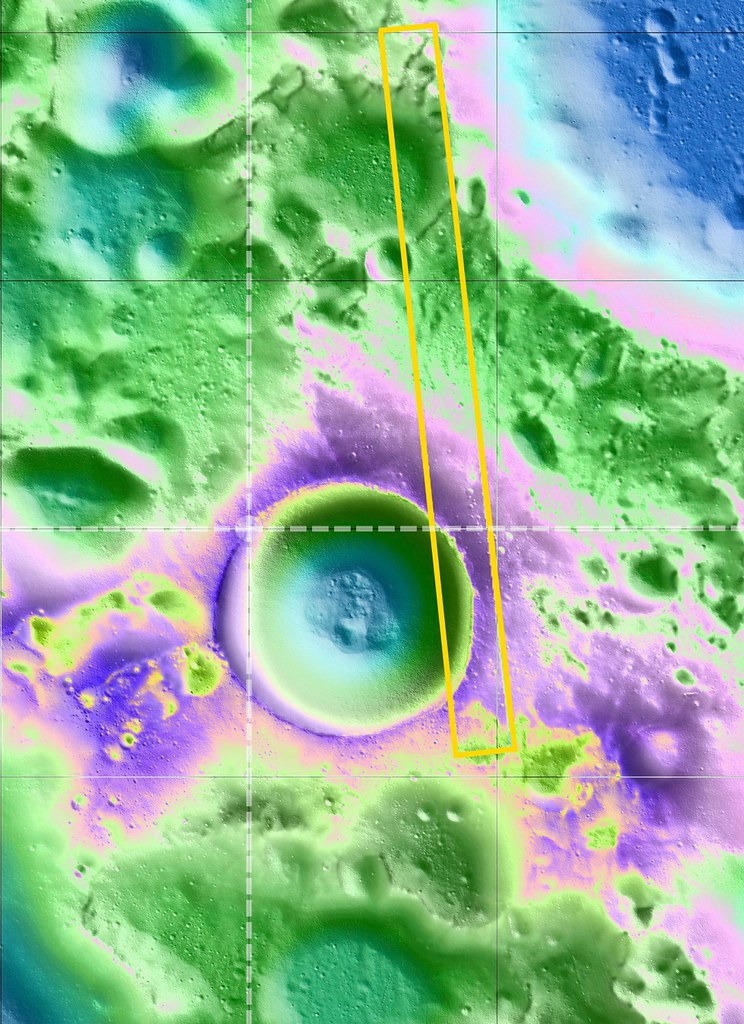 |
Quasi-permanently illuminated (and, thus, the better known areas in the vicinity of Shackleton crater and the Moon's south pole) are tinted purple on a topographic map built up from LRO's LOLA laser altimetry. The remainder of the map, including Shoemaker crater (51.8 km; 88.137°S, 45.91°E) at upper right and inside Shackleton are parts of the wide areas of the Moon now known to be permanently shadowed. The footprint of the first LROC NAC observation on June 30, 2009 is outlined in yellow [NASA/GSFC/Arizona State University]. |
Why do we care about lighting conditions at the poles? For any future long-duration missions to the lunar surface, it’s easy to see the benefit of finding a spot with near-continuous illumination to provide solar power. With that also comes a reprieve from the cold lunar night, which lasts a full two weeks at lower latitudes.
Because LRO has been in orbit for 5 years, we can now map
with great precision how much sunlight each portion of the surface sees by examining repeat imaging as the illumination from the Sun changes in the sky. In the image above, a four-km2 region on Shackleton’s rim (near the boulders) is
illuminated over 70% of the time.
LROC has shown there are no true regions of perpetual sunlight, but within small areas the surface is in sunlight nearly 94% of the year, with the longest periods of darkness lasting just 43 hours.
Over the last five years, an even bigger revolution in our understanding concerns what happens in those areas that never see the Sun – regions of true permanent shadow like the floor of Shackleton crater.
Before LRO’s launch, and really since the Apollo days, the canonical thinking was that the Moon was completely dry. There was some evidence in the form of neutron spectrometer and radar data that regions of permanent shadow may harbor water ice, but
no definitive answer. Thus many of
LRO’s instruments were designed to provide a fuller picture, so to speak, of these shadowed regions. LROC has undertaken
long-exposure images to see inside the shadows,
Diviner has measured temperatures below
-238° C (-397°F) to show a host of ices are stable,
LEND has found evidence for abundant hydrogen,
Mini-RF has shown that radar properties within shadowed regions are consistent with the presence of water ice,
LAMP and
LOLA have demonstrated that there are variations in the reflectance of materials within craters at the poles, possibly indicating ice is present at the surface.
And other spacecraft, including LCROSS, Chandrayaan-I, Deep Impact, and Cassini, have shown that hydrated materials are not necessarily limited to the poles. But as this wealth of new data has been gathered, synthesizing it into a consistent story to explain how the volatiles were deposited, migrated, and evolved over time has proved more complicated. Seemingly conflicting results (for example, LROC does not see strong reflectance contrasts within permanent shadow, but LOLA and LAMP indicate frosts may be there; LEND sees abundant hydrogen outside of regions of permanent shadow, which is a bit mysterious) have led to more questions and the need for future missions to land in these enigmatic areas. Hopefully, in a second extended mission, LRO will have the time to gather the data to give us insight to find the best landing sites.
This first image from LROC provides just one example of how our view of the Moon has been altered by LRO’s five years in orbit. Long-outstanding questions are being answered, and new questions are being raised as we examine the abundant data from LRO. This vast trove of data and wealth of new knowledge will be LRO’s legacy for decades to come.
Related Posts: