 |
Hausen's mantel? House and city block-sized boulders steadily shed down the slopes of the central peak cluster of Farside Eratosthenian crater Hausen (163.24km; 65.111°S, 271.509°E), the formation of which might have finished off excavation of the Moon's deepest vertical column (an estimated 29 km below global mean elevation), finishing off a process begun by the progenitor of the South Pole-Aitken impact basin, billions of years earlier. Hausen straddles SPA's eastern rim, where Hausen later brought to the surface materials that should include samples of the Moon's mantle, an elusive primordial layer between crust and core. Among the places to look for confirmation is this central peak high place, seen here in a 3.7 km-wide field of view from LROC NAC mosaic M1132455869LR, orbit 19007, August 29, 2013; incidence 64.65° at 49 cm resolution from 42.46 km over 64.95°S, 271.99°E [NASA/GSFC/Arizona State University]. |
Paul Spudis
The Once and Future Moon
Smithsonian Air & Space
Geoscientists want to peer ever deeper into the interior of planets. Most rocky planets have
a tripartite configuration, with a dense, metallic core at center, overlain by a rocky, iron-and magnesium-rich mantle, and finally, a low-density crust rich in silicon and aluminum on the outside. A surprise from the exploration of the Moon was that it too is configured like the Earth, with a core, mantle and crust, although of different proportions. All our samples from the Moon come only from the crust, so understanding the composition and nature of its mantle has been a high priority for lunar scientists.
With the Moon, we are fortunate to have some natural “drill holes” with which to probe the subsurface. I refer of course to the multitude of large craters on the Moon, including those largest of impact features, the multi-ring basins. Basins are impact structures hundreds of kilometers across;
the largest basin, South Pole-Aitken, is over 2500 km in diameter. Because basins are so large, they dig down many kilometers below the lunar surface. If we can identify material of deep derivation that has been thrown out during basin formation, we can
characterize the lower crust and possibly, the upper mantle of the Moon.
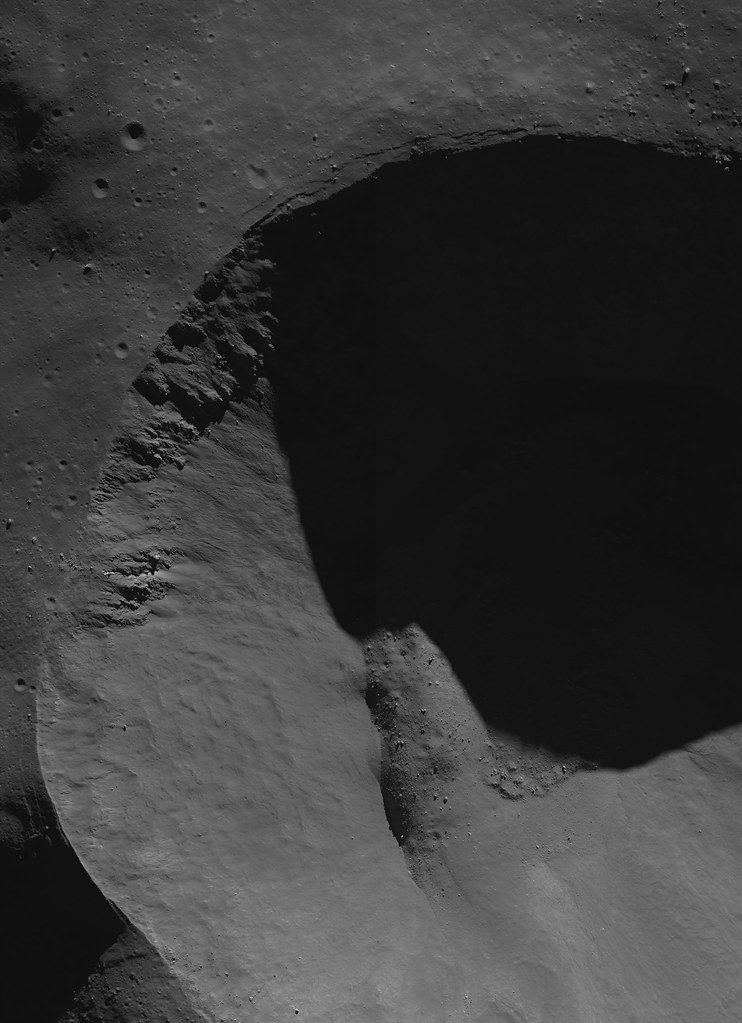 |
Copernican age excavation of Schrödinger peak ring. An unnamed 7.1 km Copernican age crater (72.13°S, 133.78°E) and excavation of the prominent Peak Rings of Schrödinger impact basin, the Moon's youngest, and itself an excavation inside much larger South Pole-Aiken impact basin, the Moon's oldest. The young crater features outcrops inside its rim that are within the 10 km "walk-back" distance from a proposed landing site. LROC NAC mosaic M126057860LR, LRO orbit 3710, April 16, 2010; 74.66° angle of incidence, 1.05 meters per pixel resolution from an altitude of 50.71 km [NASA/GSFC/Arizona State University]. |
 |
The proposed landing site (yellow ellipse), north and downslope, within 10 km "walk-back" distance from the Copernican age crater on top of the inner Schrödinger peak ring. Even at 80 meters resolution, outcrops of interest, along with other youthful anatomy in the 7.1 km crater are visible. LROC WAC observation M169698283CE (604 nm wavelength), LRO orbit 10142, September 3, 2011; incidence 72.7° [NASA/GSFC/Arizona State University]. |
Needless to say, an effort like this is fraught with difficulty. Basins are large features (at 930 km in diameter, the
lunar Orientale basin is as big as the state of Texas) and we don’t fully understand the mechanics of their formation, including such rudimentary properties as their original size (it is suspected that the final diameters of these features were enlarged by collapse, making their original size uncertain). However, that doesn't stop us from trying. Using basins as probes of the crust and mantle has been a pastime for lunar scientists for many years.
At this year’s Lunar and Planetary Science Conference (LPSC 45), the topic of the mantle was raised once again, but this time with a bit of a twist.
Decades ago,
a network of seismometers was emplaced on the Moon during the Apollo missions. This network measured the intensity and duration of “moonquakes” over the course of several years. These measurements allowed us to discover the mantle of the Moon and to estimate its density. The density is proportional to the velocity of seismic waves, which can be measured from the difference in arrival times of seismic waves at different stations for the same moonquake. From these data, we know that the mantle is composed of rock quite different in composition from surface rocks. The inferred relative density of the mantle is considerably higher – about 3.2 g/cm3 (grams per cubic centimeter; water = 1.0) than the crustal rock types (about 2.6 g/cm3). Although a single piece of information, this density constrains the mantle of the Moon to be composed of only one or two possible minerals – olivine and/or pyroxene (only these iron and magnesium-rich minerals are common enough and match the density estimate). We also suspect such mineralogy because the Earth’s mantle is made up of these minerals.
For many years, lunar scientists have searched diligently for deposits of olivine around lunar basins, material that could plausibly be interpreted as ejected from the lunar mantle. Yet to date, few such deposits have been found, and those that are seen, could just as easily derived from crustal rocks, as olivine is a common mineral in the crust as well.
 |
Crustal Thickness from GRAIL (2012) -Lunar Crust with Olivine signatures superposed over LROC WAC-DTM. Though the Moon's unexpectedly thin crust has been mapped in great detail, and constraints put on the density of lunar mantle, detection of olivine, one likely component of the crust, on the surface can't yet be definitively said to be of deep lunar origin. Full-size image HERE [NASA/JPL/MIT]. |
The recent gravity mapping of the
GRAIL mission has added confusion on this score. Assuming reasonable densities for crust and mantle, gravity maps can be interpreted in terms of crustal thickness at any given area.
The new GRAIL crustal thickness maps indicate a much thinner crust than previous estimates had shown, with a mean thickness of about 35 km, increasing to almost 45 km in some areas of thick crust. These values are about half the previous numbers and suggest that the largest impact events should have easily excavated the upper parts of the lunar mantle. The problem is that there doesn't appear to be any mantle material on the lunar surface, even proximate to the biggest basins. This absence is quite puzzling; despite the ubiquity of olivine in many lunar rocks, we do not find vast exposures of it near the rims of any lunar basin.
At the recent LPSC 45,
H.J. Melosh of Purdue University and his fellow co-workers suggested that we are looking for the wrong mineral. If the upper mantle were composed not of olivine, but a different mineral, large amounts of olivine would not necessarily be excavated by a basin-forming impact. Their calculus is as follows: the crust is thin (from GRAIL maps), computer models show that Orientale basin must have excavated the lunar mantle (from calculation), and we see pyroxene in mineral spectra of basin deposits but not olivine (from remote sensing data). Therefore, the mantle is made up of pyroxene, not olivine.
One might note that this chain of reasoning is a house of cards. IF the GRAIL measurements are telling us how thick the crust is and IF the computer models accurately reproduce basin-forming mechanics and IF the compositional data are correct, then a pyroxene mantle is required. In fact, of these ‘constraints,” only the compositional data are fact-based. GRAIL did not measure crustal thickness – it measured gravitational accelerations, objective measurements interpreted in terms of crustal thickness. Computer models attempt to simulate reality, but there is no way to directly test their validity. The scale of basin impact is so large – orders of magnitude beyond any impact event within our experience base – that unsuspected physical effects (possibly of critical importance) cannot be accounted for.
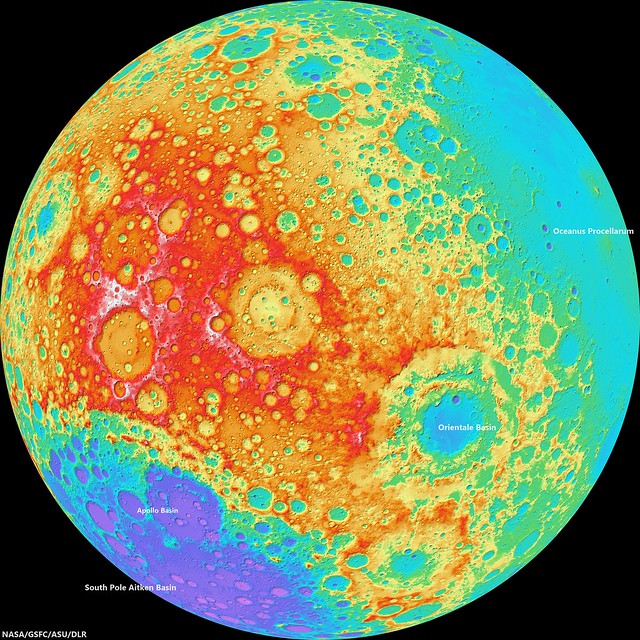 |
Though there is wide evidence that the energetic Orientale impact basin-forming event had far-reaching effects, possibly causing volcanic upwelling in widely scattered areas and the creation of strong surface magnetic fields on the direct opposite side of the Moon, it's now thought possible that the basin's progenitor might not have exposed the Moon's mantle [NASA/GSFC/Arizona State University]. |
An alternative explanation is that as large as they are, basins do not come close to digging into the Moon deeply enough to excavate the mantle. At the same session,
I presented the results of new research on the composition of ejecta from the Orientale basin, which show its deposits to be low in iron and probably derived only from upper crustal levels. This is quite surprising; the Orientale basin is one of the youngest basins on the Moon and at nearly 1000 km diameter, also one of the biggest. Virtually all of its ejecta come not only from crustal sources (as measured by remote sensing data) but also from upper crustal materials, suggesting that even the biggest impacts apparently cannot punch through the crust of the Moon.
Dr. Paul D. Spudis is a senior staff scientist at the Lunar and Planetary Institute in Houston. This column was originally published by Smithsonian Air & Space online, and his website can be found at www.spudislunarresources.com. The opinions he expressed here are his own, but these are better informed than most.